Key Takeaway
Actuators can be controlled using various methods, including manual, electric, or automated control systems. Manual control requires human operators to adjust levers or switches to direct airflow into the actuator. Electric control systems use signals to regulate the actuator’s movement by controlling the flow of air or pressure.
Automated control uses sensors and controllers to monitor and adjust the actuator’s movement based on system requirements. These methods ensure that actuators perform their tasks accurately, reliably, and efficiently in systems like robotics, manufacturing, and fluid control.
Manual Control Systems
Manual control systems are the simplest form of controlling pneumatic actuators. In these systems, operators manually adjust the valves to direct compressed air into the actuators, causing them to perform their required motions. These systems are typically used in smaller-scale applications or where automation is not yet feasible. While manual control offers direct and immediate operation, it is labor-intensive and can be prone to human error.
In industrial settings, manual control is often used in tandem with more advanced automation systems to provide operators with the ability to override or adjust the system as needed. For instance, operators may manually control the flow of air into the system when precise adjustments are needed or during troubleshooting and maintenance activities.
Despite the convenience of manual control, it can be less efficient compared to fully automated systems. For high-volume or repetitive tasks, manual systems often become impractical, leading industries to shift toward automated control solutions. However, for some niche or smaller operations, manual control systems continue to play a role in managing pneumatic actuators effectively.
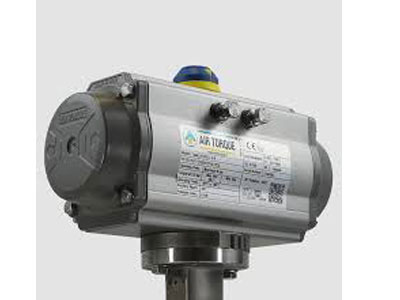
Automated Actuator Control Mechanisms
Automated actuator control systems have revolutionized the way industries operate by allowing precise, reliable, and uninterrupted actuator movement. Unlike manual systems, automated control uses sensors, actuators, and electrical signals to control the movement without human intervention. These systems rely on controllers and programming to execute predefined tasks.
One of the most common automated systems involves using proportional, integral, and derivative (PID) controllers, which continually monitor the actuator’s position and speed. Automated systems also employ feedback from sensors to adjust force and direction in real-time, ensuring that the actuator performs precisely as required.
Pneumatic actuators are often controlled by solenoid valves and pressure regulators in an automated setup, while electric actuators use motor controllers for precise movements. In these systems, the main advantage is efficiency, as they can perform tasks repetitively without fatigue, reducing human error and improving safety by minimizing operator involvement.
Automated control also allows for complex actuation sequences, such as sequential movements of multiple actuators, which would be difficult or impossible with manual systems. Moreover, these systems are adaptable to real-time changes, making them ideal for high-speed and high-precision operations across industries like automotive manufacturing, robotics, and aerospace. Ultimately, automated systems increase productivity and consistency while reducing the need for manual labor.
You May Like to Read
Feedback Loops for Precision Control
Feedback loops are essential for ensuring the precise and accurate control of actuators in complex systems. A feedback loop is a system where information about the actuator’s performance (such as position, speed, or force) is continuously sent back to the controller, which uses this data to adjust the actuator’s behavior.
In pneumatic or hydraulic actuators, position sensors are often employed to track the movement of the actuator’s piston. The sensor provides real-time feedback, such as the distance the actuator has traveled. Based on this data, the controller can adjust the air pressure or flow rate to ensure the actuator reaches its target position without overshooting. Similarly, force sensors or velocity sensors provide additional data to ensure the actuator is performing within the required force and speed parameters.
The primary goal of feedback loops is to maintain the system’s accuracy and precision. These loops enable the actuator to make real-time adjustments, compensating for external factors such as temperature fluctuations, friction, or load changes that could affect its performance.
For instance, a closed-loop feedback system ensures the actuator moves only as much as needed, preventing over-extension or failure. These systems are particularly important in applications that require high precision, such as robotics, automated manufacturing, and medical devices. They help reduce errors, improve the lifespan of actuators by minimizing wear, and ensure optimal performance in dynamic environments.
Role of Programmable Logic Controllers (PLCs)
Programmable Logic Controllers (PLCs) are an essential part of modern actuator control, offering a high degree of automation and precision in industrial systems. A PLC is a digital computer used for automating complex industrial processes, including the control of actuators. It receives input signals from sensors or switches, processes the data based on programmed instructions, and sends output commands to actuators to control their movement.
The PLC’s primary role is to execute control algorithms and logic in real-time, allowing for seamless automation of actuator-driven processes. For example, in a manufacturing line, the PLC can control a series of actuators to move parts from one station to the next, based on pre-programmed schedules or sensor data. It ensures that the actuators move at the right time and in the correct sequence, facilitating high-speed automation and reducing errors.
One of the key benefits of using PLCs in actuator control is their flexibility. PLCs can be easily reprogrammed to adapt to changing production needs or different actuator configurations, making them ideal for industries with varying tasks. They also support integration with other systems, such as SCADA (Supervisory Control and Data Acquisition) and HMI (Human-Machine Interface), allowing for centralized control and monitoring of actuator systems.
In short, PLCs offer a high level of control, efficiency, and reliability. They are widely used in sectors like automated manufacturing, robotics, and process control, where precise control of actuators is critical to the system’s operation.
Advancements in Wireless and IoT-Based Control
The rise of wireless control and Internet of Things (IoT)-based technologies has significantly advanced actuator control, offering greater flexibility, remote access, and real-time monitoring. These innovations allow operators to control actuators and monitor their performance from virtually anywhere, without the need for wired connections.
Wireless actuators use technologies such as Bluetooth, Wi-Fi, or Zigbee to communicate with control systems, enabling remote operation. These systems eliminate the need for long, costly wiring and allow for easier integration of actuators into complex industrial environments. For example, a maintenance technician can monitor and control actuators in a factory from a tablet or smartphone, improving efficiency and response times.
On the other hand, IoT-based control systems take actuator control to the next level by connecting devices to the cloud and enabling data sharing between machines. This data-driven approach allows for predictive maintenance, where sensors embedded in actuators relay data on their health and performance. This data can then be analyzed to detect any signs of wear or failure before they lead to major problems, allowing for proactive maintenance.
IoT integration also enables real-time feedback on actuator performance. For instance, an actuator’s position, force, and speed can be monitored and adjusted remotely to ensure optimal performance. This level of control reduces downtime, enhances automation, and improves operational efficiency in applications across industries like manufacturing, logistics, and energy management.
Overall, wireless and IoT-based control represents the future of actuator control, offering unmatched flexibility, scalability, and efficiency in a connected world.
Conclusion
In conclusion, actuators can be controlled using various methods, including manual, electrical, hydraulic, and pneumatic systems. Each method provides distinct advantages depending on the application requirements, offering flexibility in selecting the most suitable control mechanism for specific tasks.
Additionally, advances in technology have led to the development of sophisticated control systems that integrate sensors and feedback loops, ensuring precise and efficient actuator operation. These methods enable seamless automation processes, enhancing productivity and performance across different industries. As automation demands continue to grow, the ability to tailor actuator control methods to specific needs remains a critical factor in optimizing operational efficiency and achieving desired outcomes in industrial applications.